Congenital pulmonary airway malformation (CPAM) is a benign tumor of the lung characterized by a cystic mass of disorganized but otherwise normal lung tissue. It is thought to occur from abnormal development of the embryonic lung bud at the fifth or sixth week of development. CPAMs can occur in any lobe of the lung and rarely affects more than 1 lobe. CPAM is slightly more common in males and rarely presents before 20 weeks of gestation.
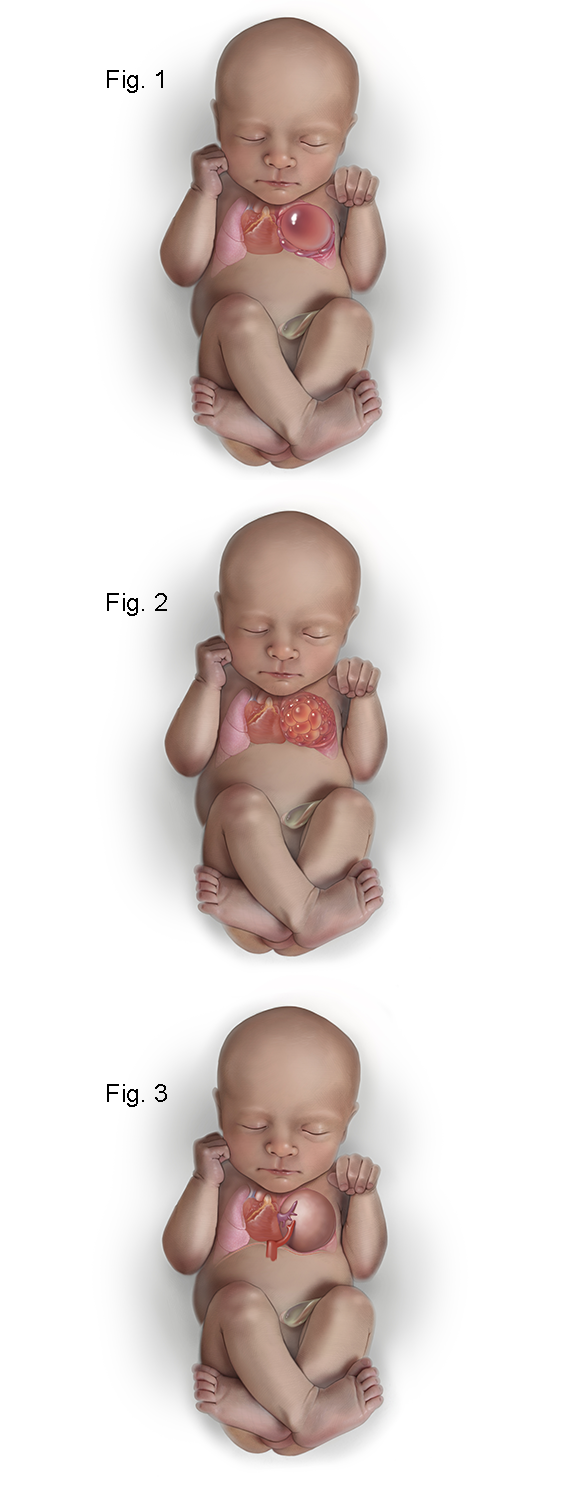
CPAMs are usually diagnosed on routine prenatal ultrasound, but rarely before 20 weeks gestation. After 20 weeks gestation, the CPAM grows very rapidly. There is a classification system that divides CPAM into 5 categories:
- Type 0 represents 1 to 3% of cases in which small cysts cover almost all of the lungs, and this type is incompatible with life.
- Type I CPAMs account for 50% of cases and have relatively few large cysts, ranging in size from 3 to 10 cm. Figure 1 shows the fetus with a left upper lobe type I congenital pulmonary airway malformation that has a large unilocular cyst which is shifting the heart and mediastinum to the left and compressing the left lower lobe.
- Type II CPAMs account for 40% of cases and have numerous cysts of smaller size, usually less than 1 cm. Type II CPAMs are associated with multiple congenital anomalies. Figure 2 shows the fetus with a type II CPAM in which the cysts are smaller than in type I.
- Type III CPAMs are usually large homogeneous, solid-appearing masses, which can grow to large sizes in utero and result in nonimmune hydrops. Figure 3 shows a type III CPAM which is microcystic and on ultrasound appears solid. In addition, there is a systemic arterial feeding vessel making this a “hybrid” CPAM which has features of both a CPAM and a bronchopulmonary sequestration (BPS).
- Type IV CPAMs account for 15% of cases and are characterized by very large cysts, up to 10 cm in size.
CPAMs are rarely diagnosed prior to 20 weeks gestation after which they grow quite rapidly reaching a peak at an average gestational age of 26 weeks. The growth of the CPAM then reaches a plateau after which it gradually decreases in size. The normal fetal lung tissue and the baby grow around the CPAM. During the rapid phase of growth, the CPAM can become quite large. This may shift the heart and mediastinum, invert the diaphragm, and cause nonimmune hydrops (fluid collections in the chest, abdomen, skin edema, and swelling of the placenta). The CPAM volume ratio (CVR) helps predict which CPAMs will be at increased risk for developing hydrops. The CVR estimates the volume of the CPAM using the formula for a prolate elipse (length x width x height x 0.52) divided by the head circumference. A value at presentation of < 1.6 is associated with a favorable outcome with a 2% risk of hydrops. (Hydrops occur in type I and type IV CPAMs with large dominant cysts, which acutely increase in size secondary to rapid fluid accumulation.) In contrast, CPAMs with CVR > 1.6 at presentation have an 80% risk of progressing to hydrops.
At 32 to 34 weeks gestation, there are changes in the number of fetal alveoli and the fluid within the lung. At this gestational age, CPAMs, especially type III CPAMs, become sonographically invisible. The CPAM is still present but is sonographically indistinguishable from adjacent normal lungs. If a fetal MRI is obtained, these “disappearing CPAMs” show up brightly in contrast to the normal lung.
It is important to distinguish low-risk and high-risk CPAMs based on the CVR. CPAMs with CVR < 1.6 rarely get into trouble and only need to be followed on a weekly basis until a plateau is reached at approximately 26 weeks gestation. In contrast, a CPAM with a CVR > 1.6 is at markedly increased risk for the development of hydrops. In these cases, we treat mothers with two intramuscular injections of betamethasone, a steroid that helps arrest the rapid growth of the CPAM. In high-risk CPAMs with a CVR > 1.6 and no hydrops, treatment with prenatal steroids has been associated with 100% postnatal survival. In high-risk CPAMs with CVR > 1.6 and hydrops, steroids may still be effective with 40% postnatal survival. In addition, these hydropic CPAMs may respond to additional courses of betamethasone if hydrops do not resolve after the first course.
In cases unresponsive to betamethasone, fetal surgery may be indicated. Cases with a large cyst may benefit from thoracoamniotic shunt placement. In some CPAM cases, fetal thoracoscopy may be used to create communications between all the cysts within the CPAM to maximize the effect of the thoracoamniotic shunt that is used to decompress it (see Figures 4-7 below).
Figure 4. The illustration shows the fetoscope has been inserted into the fetal chest and into the dominant cyst of the CPAM with a laser fiber (green tip) extended at the end of the fetoscope for laser fenestration of non-communicating cysts in the CPAM.
Figure 5. The illustrations shows a close up of the laser fiber extended from the tip of the fetoscope in order to create a communication with the dominant cyst of non-communicating cyst by using laser energy to burn a hole in the cyst wall.
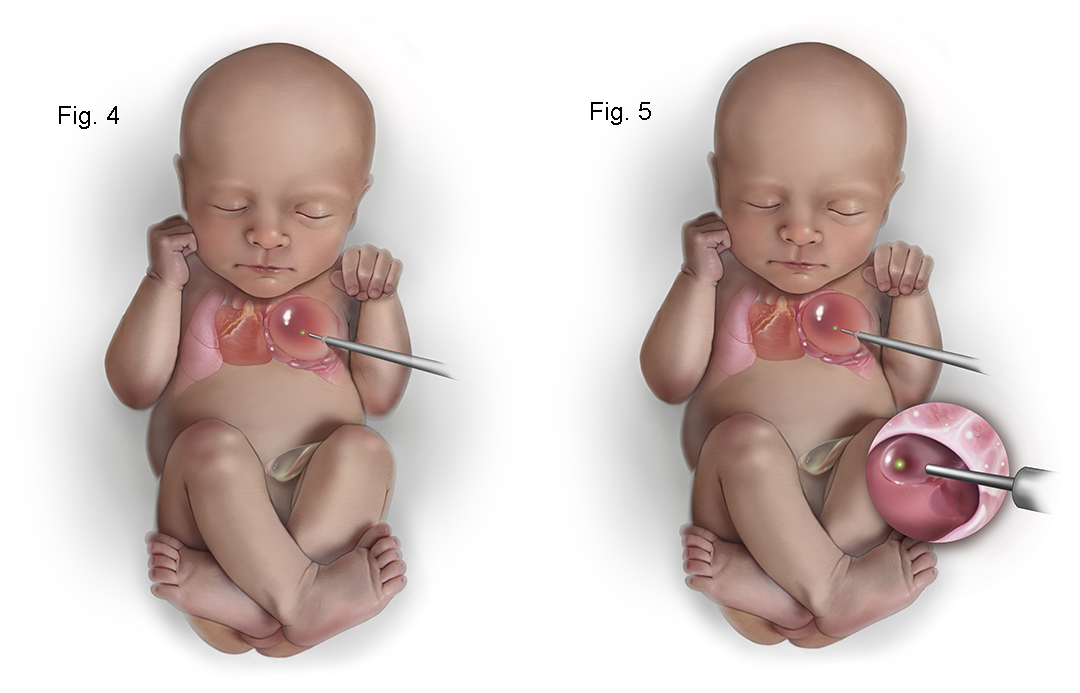
Figure 6. The illustration shows a close up view of the internal CPAM showing communication of the cysts with the dominant cyst allowing more complete decompression of the CPAM.
Figure 7. The illustration shows the placement of a thoracoamniotic shunt with a double pigtail catheter which allows drainage of fluid within the CPAM to the amniotic cavity. Internally the shunt sits with a pigtail within the CPAM and it passes through the chest wall with a pigtail on the outside to drain fluid into the amniotic cavity.
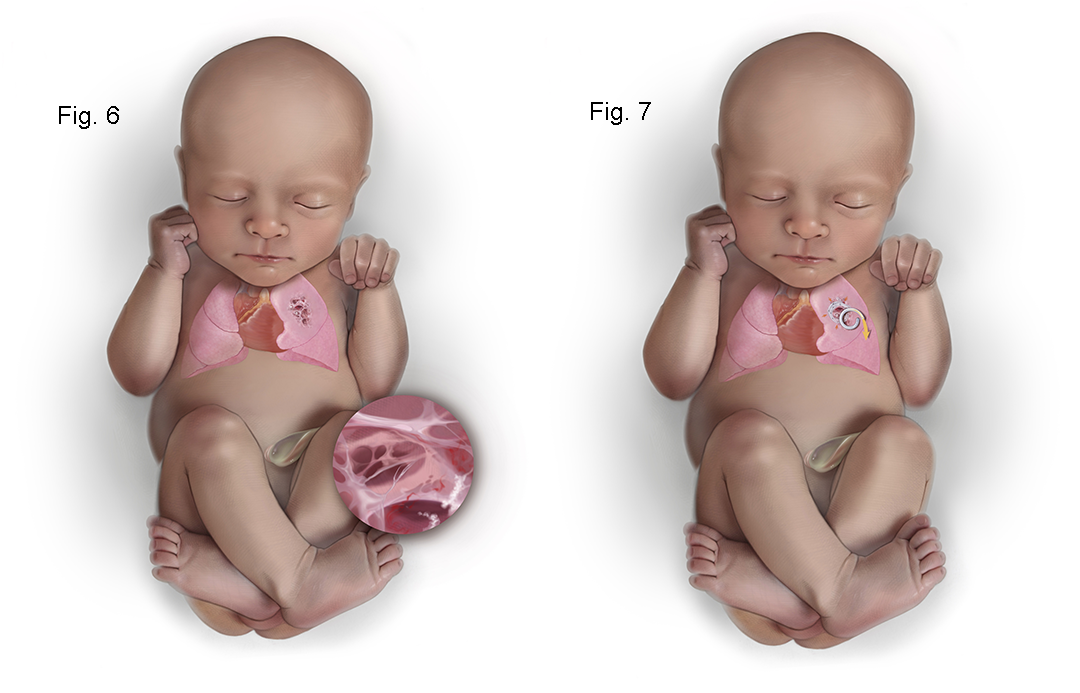
In solid type III CPAMs with hydrops, shunting is of no benefit. If unresponsive to steroids, the only option may be open fetal surgery for thoracotomy and lobectomy. This is rarely necessary with the use of steroids, but open fetal surgery has a 65% survival rate. A newer less invasive approach is ultrasound-guided intravascular laser photocoagulation (see Figures 8-9 below). The lobar pulmonary artery to the lobe with a CPAM is coagulated causing regression in the size of the CPAM. This allows the baby to grow around the CPAM. This approach does not sacrifice any lung function because the CPAM provides no functional gas exchange after delivery.
Figure 8. The illustration shows how a type III hybrid CPAM can be treated with intravascular laser photocoagulation. An 18 gauge needle is passed under ultrasound into the CPAM into the systemic feeding vessel and the laser fiber is inserted into the vessel just beyond the tip of the 18 gauge needle. Turing on the laser fiber uses light to coagulate the systemic feeding vessel which prevents the CPAM from growing and causes it to regress in size.
Figure 9. The illustration shows the 18 gauge needle in the systemic feeding vessel with the laser fiber extended into the vessel lumen about to be fired to photocoagulate the vessel.
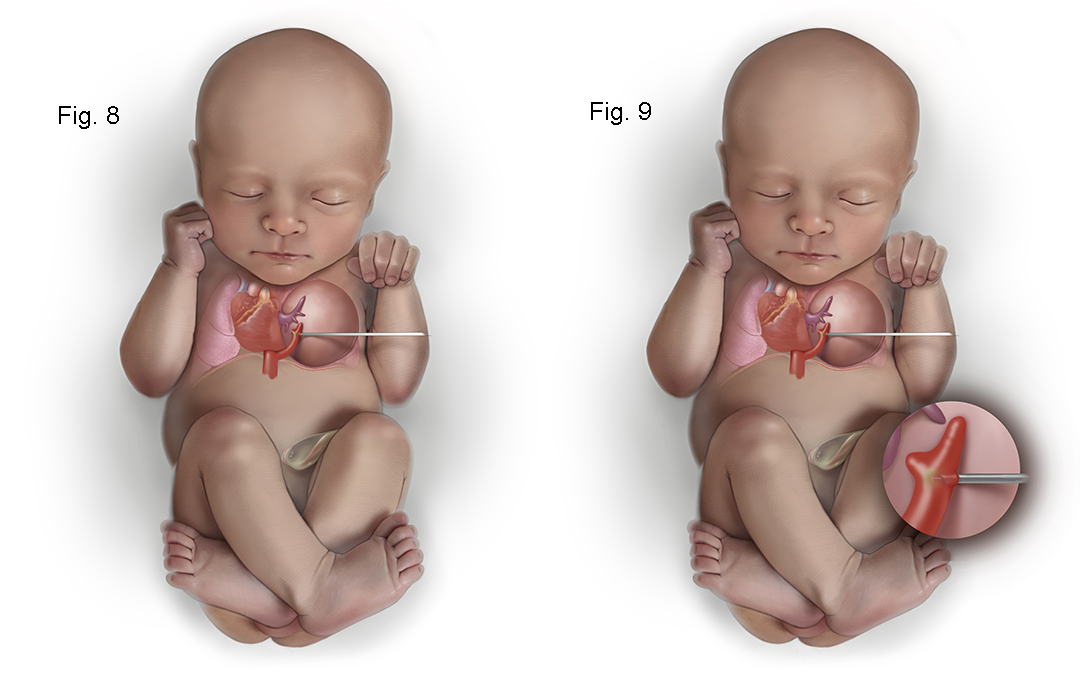
In cases in which the CPAM is asymptomatic, workups can be deferred to outpatient follow-up. If the CPAM is symptomatic, whether due to tachypnea, perioral blueness, or respiratory distress, the baby should have surgery urgently to remove the CPAM. It is usually apparent which CPAMs are likely to get into trouble after delivery due to CVR > 1.6 or the presence of large cysts at risk for “air trapping” (cysts fill with air but cannot exhale, trapping air in the CPAM which progressively larger). In asymptomatic CPAMs, we obtain a high-definition thin-section CT scan of the chest with IV contrast when the baby can have IV sedation without the need for hospital admission for apnea monitoring. In full-term infants, this is after 45 post-conceptual weeks of age, but if the baby was born less than 36 weeks gestation, this will need to wait until after 55 post-conceptual weeks of age.
A CT scan has several important roles in decision-making regarding CPAMs. First, it can confirm the diagnosis of CPAM and rule out segmental lobar emphysema, which can mimic type III but does not require surgery. Secondly, a CT scan can identify systemic feeding vessels, which occur in hybrid CPAMs and have features of both CPAM and bronchopulmonary sequestration. Lastly, a CT scan helps determine if a lung-sparing operation can be performed, or if a formal lobectomy will be necessary. For example, a CPAM located in the periphery of the lung can often be stapled or wedged out preserving most of the normal lung tissue in that lobe. In contrast, a CPAM that is centrally located may require lobectomy, as the removal of the CPAM may compromise either the arterial perfusion or bronchial connection with the peripheral tissue in the lobe.
It is controversial whether all CPAMs need to be removed. Certainly, all symptomatic CPAMs should be removed, and this is a uniformly accepted practice. In asymptomatic CPAMs however, opinions vary widely. Some centers believe that surgery for CPAMs should be deferred until complications arise. Once complications occur, however, the risks of the surgery go up, and if a malignant transformation has occurred, the mortality increases.
Dr. Crombleholme’s approach to the question of whether all CPAMs should be removed is informed by 35 years of experience with evaluating and operating on over a thousand prenatally diagnosed CPAMs. This is a question of risk-to-benefit ratio. There are several risks to leaving CPAMs including:
- Infection: The CPAM has an anomalous connection to the airway and lacks clearance mechanisms to fight infection, which can occur in up to 5 to 10% of cases
- Malignant Transformation: Malignant tumors can arise within the CPAM (bronchioloalveolar carcinoma, rhabdosarcoma, cystic bronchopulmonary blastoma). This usually takes three to four decades, but 10% of all malignant tumors removed in patients under 20 years of age were noted to arise from CPAMs. The youngest patient ever reported with a malignant tumor arising in a CPAM was only 14 months of age.
- Long-Term Follow-Up: If you remove the CPAM, the child doesn’t need long-term follow-up, but if you leave the CPAM, you commit the child to lifetime follow-up hoping to identify a complication before it occurs.
Taking out the CPAM, which is a nonfunctional lung tissue, allows compensatory growth of the normal lung once the mass is removed. In his personal experience, Dr. Crombleholme has no mortality in minimal morbidity with resection of CPAMs with an average postoperative stay of only 48 hours.
Minimally invasive surgery, either by thoracoscopic resection or muscle-sparing thoracotomy, is the best option. They avoid complications such as scoliosis and limitations in shoulder range of motion seen with standard posterolateral thoracotomy. Thoracoscopic surgery uses multiple ports, usually 5 mm in size, to dissect and remove the CPAM. The instruments used for thoracoscopic surgery were not designed for use in infants and at least one port must be enlarged to remove the CPAM. A muscle-sparing thoracotomy is performed through a 3 cm incision in the axilla and the muscles on the chest wall (latissimus dorsi and serratus anterior) are not divided and only the intercostal muscles between the ribs are cut in gaining access to the chest. This is performed with a thoracic epidural for postoperative pain control with the baby taking the bottle or breast the night of the surgery, the chest tube removed on post-op day 1, and discharge home on post-op day 2.
In Dr. Crombleholme’s experience with close to 1000 CPAM sections, there has been no mortality and virtually no morbidity. These children do well long-term with a slight increase in the incidence of asthma but have no exercise limitations and excellent pulmonary function.
Would you like to schedule an appointment with our Fetal Care Center?
For Medical Professionals
Congenital cystic adenomatoid malformation (CCAM), or the more recent term congenital pulmonary airway malformation (CPAM) of the lung, is a lesion characterized by a multicystic mass of pulmonary tissue with a proliferation of bronchial structures (1,2). It may represent a failure of maturation of bronchiolar structures, occurring at approximately the 5th or 6th week of gestation, during the embryonic stage of lung development (1-3). Alternatively, it may represent focal pulmonary dysplasia, since skeletal muscle has been identified within the cyst walls (4). Others have suggested that it may be the result of airway obstruction (5-8). The gestational age and location of the airway obstruction may determine whether CPAM, bronchopulmonary sequestration, or lobar emphysema results (9,10).
CPAM is slightly more common in males and may affect any lobe of the lung (11). The lesion is unilobar in 80% to 95% of cases and bilateral in fewer than 2% (1). Unlike bronchopulmonary sequestration (BPS), CPAMs have communication with the tracheobronchial tree, albeit via a tortuous connection. In contrast to BPS, CPAMs usually derive their arterial blood supply and venous drainage from normal pulmonary circulation, but anomalous arterial and venous drainage of CPAM have also been reported (12) as well as the so-called “hybrid” CPAMs that have a systemic blood supply (13).
Stocker et al., originally subdivided CPAM into three types based on their pathologic characteristics (1). More recently, Stocker revised this classification to include five types. Stocker recommended that types 0 and IV CPAMs be added to types I, II, and III (1,14). The five types were intended to represent the spectrum of malformations of five successive groups of airways. Type 0, a condition previously described as acinar dysplasia (15) is now described as bronchial; type I as bronchial/bronchiolar; type II as bronchiolar; type III as bronchiolar/alveolar duct; and type IV as peripheral. Because of the broad spectrum of malformations covered by this expanded classification system, Stocker first suggested the term congenital pulmonary airway malformation (CPAM) as it better described the nature of the spectrum of lung lesions, which is now the preferred term (14). Stocker’s classification is a histologic one, although commonly applied to prenatal sonographic appearance.
Type 0 CPAMs are quite rare accounting for only 1-3% of cases and is incompatible with life. The lungs are diffusely involved and appear small. Histologically, type 0 CPAMs consist of bronchiole-like structures with muscle, glands, and numerous cartilage plates. There are small cysts of 0.5 cm with ciliated pseudostratified epithelial lining and goblet cells, and bronchiolar cartilage involving all lobes (14).
Type I lesions account for 50% of postnatal cases of CPAM, and consist of single or multiple cysts lined by ciliated pseudostratified epithelium. These cysts are usually quite large (3–10 cm) and few in number (1–4) (16). Type I lesions are usually associated with a favorable outcome.
Type II lesions account for 40% of postnatal cases of CPAM and consist of more numerous cysts of smaller diameter (usually less than 1 cm) lined by ciliated, cuboidal, or columnar epithelium. Respiratory bronchioles and distended alveoli may be present between these cysts. There is a high frequency of associated congenital anomalies with type II lesions (16,17). The prognosis for type II lesions often depends on the severity of associated anomalies. The most commonly associated anomalies include genitourinary, such as renal agenesis or dysgenesis; cardiac, including truncus arteriosus and tetralogy of Fallot; jejunal atresia; diaphragmatic hernia; hydrocephalus; and skeletal anomalies (1). The high incidence of associated anomalies has led to speculation that this type of CPAM may occur as a result of events occurring prior to 31 days of gestation (18).
Type III lesions account for only 10% of cases and are usually large homogeneous microcystic masses that cause mediastinal shift. These lesions have bronchiole-like structures lined by ciliated cuboidal epithelium, separated by masses of alveolarsized structures lined by nonciliated cuboidal epithelium (16). The mixture of epithelial and mesenchymal structures in type III lesions has led to speculation that their development is related to events occurring prior to 28 days of gestation, after the formation of the lung buds (18). The prognosis in type III CPAMs is variable but can, in severe cases, present with nonimmune hydrops in utero and cardiorespiratory compromise in the newborn (19,20).
Type IV CPAMs account for approximately 15% of cases and are characterized by very large cysts up to 10 cm lined by flattened epithelium resting on loose mesenchyme (16,21,22. These lesions can have areas of focal stromal hypercellularity with histologic overlap with grade I pleuropulmonary blastoma (23,24).
Adzick et al., proposed a simple modification of Stocker’s classification of CPAMs to sonographic appearance to assist in prenatally predicting outcome (19). In this classification, macrocystic CPAMs have single or multiple cysts > 5 mm in diameter. Microcystic CPAMs are more solid and bulky, with cysts that are < 5 mm in diameter. This distinction can easily be made sonographically in the fetus. Macrocystic lesions appear sonographically as fluid-filled cysts, while microcystic lesions appear solid with an almost homogeneous appearance (19). This is a useful sonographic distinction, because microcystic lesions are at increased risk for the development of hydrops. The high mortality rate of microcystic lesions is due to the large size these lesions attain and secondary sequelae, including mediastinal shift, pulmonary hypoplasia, polyhydramnios, and nonimmune hydrops (19,20).
The differential diagnosis of fetal thoracic masses includes congenital diaphragmatic hernia (CDH), bronchogenic or enteric cysts, BPS, mediastinal cystic hygroma, bronchial atresia or stenosis, and neuroblastoma (25-29). The sonographic appearance of CPAM will influence the differential diagnosis. Type I CPAMs are more likely to be confused with a CDH. Observing peristalsis in the loops of herniated intestine or emptying of the stomach herniated through the diaphragm may help to differentiate the two (30).
Fetal magnetic resonance imaging (MRI) may be helpful in evaluating fetal chest masses and distinguishing them from diaphragmatic hernia (31). It is also worth noting that CPAM can coexist with CDH (1). The microcystic type III CPAMs are highly echogenic. This is helpful in distinguishing CPAM from solid tumors such as neuroblastoma. Bronchogenic cysts are unilocular and are usually adjacent to major bronchi, which may be confused with a type I CPAM. However, the main differential diagnosis in type III CPAM is usually BPS. Unlike most CPAMs, BPS derives its blood supply from the systemic circulation (32). This systemic blood supply to BPS can often be demonstrated with the use of color flow Doppler studies (33,34). The demonstration of systemic blood supply to a thoracic mass has been thought to be pathognomonic of BPS. More recently, Cass et al., described six cases of cystic adenomatoid malformation that had systemic blood supply (13). These lesions were called “hybrid” lesions as they had features of both CPAMs and BPSs and their natural history was also a mixture of the two lesions. The prognosis in hybrid CPAM is more favorable than “pure” CPAM (35).
CPAM has been considered a relatively rare lesion, although in more recent years, with the widespread use of obstetrical ultrasound, there has been a rapid increase in the number of cases detected prenatally (36,37). A commonly quoted incidence of CPAM lesions was between 1 in 25,000 and 1 in 35,000 livebirths (38), but a more recent estimate of the incidence at 0.87 to 1.92 in 10,000 live births is probably more accurate (39).
CPAM is diagnosed by prenatal ultrasound demonstrating a lung tumor that may be solid or cystic, and with an absence of systemic vascular flow (40-47). Types I and II CPAM appear as cystic or echolucent pulmonary masses, and may appear similar to diaphragmatic hernia, cystic hygroma, and other cystic lesions, such as bronchogenic or enteric cysts, and pericardial cysts (48). In contrast, type III CPAM typically appears as a large hyperechogenic mass, often associated with mediastinal shift and, in advanced cases, hydrops (25).
The sonographic appearance of CPAMs can range from a solid echo dense mass filling the chest to a lesion with a single dominant cyst with a compressive effect on the mediastinum. The vast majority of CPAMs derive their blood supply from the pulmonary circulation and drain via the pulmonary veins. However, color Doppler should be used to search for the presence of a systemic feeding vessel. This may be observed in most BPSs (the main differential diagnosis in CPAMs) and in “hybrid” CPAM lesions (13). The systemic feeding vessel in hybrid CPAM lesions usually comes directly off the descending aorta; however, transdiaphragmatic systemic feeding vessels have also been observed in hybrid CPAMs.
A change in the echogenicity of type III CPAMs may occur between 30 and 34 weeks in which they become isoechogenic with the adjacent normal lung. Although sonographically invisible, such cases of CPAM are still readily apparent on MRI. Occasionally, postnatal imaging with CT scanning reveals no evidence of CPAM, which may be due to the presence segmental or lobar hyperinflation of otherwise normal lung parenchyma.
The normal fetal lungs have a homogeneous appearance with moderately high signal intensity on T2-weighted images (49). The T2 signal intensity increases with gestational age and T1 signal intensity decreases with gestational age. This effect is thought to be secondary to the increasing number of alveoli as gestation progresses as well as increasing lung fluid production (50). In the setting of a CPAM, the compression of the normal lung by the mass of the CPAM may make the adjacent normal lung appear lower in signal intensity on T2 images compared to the contralateral normal lung (49). The liver, mediastinal structures, and lung are easily differentiated from each other by their signal intensity.
Fetal MRI is a valuable adjunct to ultrasound in the evaluation of CPAMs. It is often not possible to determine which lobe is involved in the CPAM by ultrasound. In contrast, fetal MRI is often able to delineate the T2 signal intense CPAM from compressed normal lobes which have a lower T2 signal compared to normal lungs. Fetal MRI has proven useful when the diagnosis based on ultrasound alone is uncertain and increases the specificity of the diagnosis (51-53).
Macrocysts within CPAMs are highly signal intense of T2 weighted images with adjacent solid components of the CPAM also being high on T2-weighted images. CPAMs that are entirely macrocystic are hyperintense on T2. Microcystic CPAMs may be difficult to distinguish from lobar or segmental hyperinflated lung. The presence of cysts confirms the diagnosis of CPAM, but in the absence of macrocysts, it may be difficult to distinguish a hyperinflated lung from a type III CPAM. The vascular distribution in a hyperinflated lobe or segment is normal, whereas in a CPAM, the normal vascular distribution is disrupted by irregular spacing of vessels by signal intense parenchyma. It may not be possible to distinguish microcystic or type III CPAMs from hyperinflation on prenatal MRI and postnatal thin-section CT scans with intravenous contrast may be needed to make this distinction with certainty. It may also be difficult to distinguish a microcystic hybrid CPAM with a systemic arterial feeding vessel from an intralobar bronchopulmonary sequestration. Both BPS and a type III CPAM appear homogeneously signal intense on T2 weighted images and both will have a flow void from the systemic feeding vessel. A concomitant pulmonary arterial feeding vessel can be seen in hybrid CPAMs as well as intralobar BPS.
It is not well established what is the optimal timing in gestation to image CPAMs. Few CPAMs present prior to 20 weeks gestation, and most MRIs are obtained in the late second trimester at the time a diagnosis is made or suspected on prenatal ultrasound. Some have suggested that repeat MRI in late gestation improves diagnostic accuracy and may reduce the need for postnatal imaging. Late third-trimester fetal MRI may be helpful in deciding whether or not an EXIT-to-Resection may be indicated. While there is no consensus about the indications for the EXIT procedure for CPAMs, it is thought that a shift of the mediastinum, causing complete effacement of the intrathoracic trachea, would be an indication for the EXIT procedure (54). This would be difficult to determine by ultrasound in the later third trimester as there is usually less amniotic fluid, and the acoustic windows for imaging the fetal chest are limited.
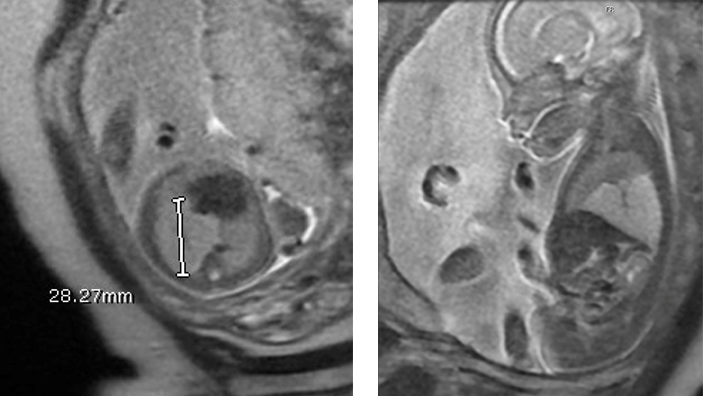
There have been several MRI-derived quantitative measurements reported to predict the risk of developing hydrops and postnatal respiratory compromise. MRI-specific quantitative measurements include the fetal MRI-derived lung mass volume ratio (LMVR) (which is the MRI equivalent of the CVR), the lesion-to-lung volume ratio (LLV), and the observed/expected normal fetal lung volume (O/E-NFLV) (55). Of these MRI-derived measurements, the LMVR was found to be the strongest predictor of the development of hydrops and neonatal respiratory distress after delivery. But there was no direct comparison made between LMVR and CVR. While LMVR is measured by the sum of serial coronal MRI images, CVR is measured by 3-dimensional measurements obtained by ultrasound and normalized for gestational age by dividing by the head circumference. One would predict that LMVR would be equivalent to CVR as they are measuring the same thing using different modalities.
Although in the majority of cases, CPAMs are isolated lesions, in 10-20%, particularly in type II lesions, there can be associated anomalies as noted above (56,57). MRI can be particularly helpful in excluding these anomalies or defining in fetuses known to have a CPAM.
The postnatal natural history of CPAM can be quite variable (36,58). The lesion can be completely asymptomatic and come to medical attention only when chest radiography is performed for other reasons, such as a history of mild respiratory complaints or recurrent infections in infancy or childhood. However, most postnatal patients will present at birth with severe cardiorespiratory compromise due to severe pulmonary hypoplasia (1,33,59-65). Even before the advent of obstetrical sonography, it was recognized that up to 14% of cases of CPAM result in stillbirths (1). This observation hinted at the different prenatal natural history of CPAM.
Our understanding of the natural history of CPAM is still evolving. The worst outcome is observed in fetuses in which hydrops develops (19,20,66). Hydrops is usually seen in very large lesions, often type III, which cause mediastinal shift and vena caval obstruction. Hydrops may also be exacerbated by the loss of protein from the CPAM into the amniotic fluid, thus reducing the fetal colloid oncotic pressure from hypoproteinemia (33). Anecdotal reports exist of fetuses with CPAM surviving after the onset of hydrops (67-75). Diamond et al., suggested that resolution by 30 weeks’ gestation may be more common than is appreciated (76). The reason for this unexpected resolution of hydrops in CPAM was not apparent until the natural history of CPAM was defined by Crombleholme (35). CPAMs plateau in their growth at an average of 26 weeks’ gestation, after which the fetus grows around the CPAM allowing hydrops to resolve (35).
The overall prognosis depends primarily on the size of the lesion, as well as whether it is predominantly macrocystic or microcystic. Polyhydramnios is seen in up to 70% of CPAMs diagnosed antenatally (36). The pathogenesis of polyhydramnios is not completely understood but is thought to relate to esophageal obstruction from mediastinal shift and interference with fetal swallowing of amniotic fluid (2,77). This is supported by the absence of fluid in the fetal stomach in such cases.
The diagnosis of CPAM may also have implications for the health of the mother. The mother with a fetus with CPAM may develop the “mirror syndrome,” a hyperdynamic preeclamptic state that may be life-threatening. The “mirror syndrome” has also been seen in molar pregnancies, sacrococcygeal teratoma, and in fetal conditions that result in poor placental perfusion, which leads to endothelial cell injury (78,79). The only treatment for this syndrome is immediate delivery of the baby and placenta.
The antenatal diagnosis of a large CPAM might at first appear to be an ominous finding; however, several older reports have described disappearing fetal lung masses (58,66,80-83). MacGillivray et al., have reported six cases of large CPAM with associated mediastinal shift that progressively decreased in size over the course of gestation (83). These lesions were all of the microcystic, or type III, variety but none was associated with hydrops. It is now believed that these “disappearing” CPAMs are an artifact of changing echogenicity of the lung at 32-34 in which normal lung becomes isoechogenic with the CPAM. If an MRI is obtained, the CPAM is readily distinguished from normal lung on T2 weighted images despite having “disappeared” by ultrasound.
One of the largest experiences with prenatally diagnosed CPAMs was reported by Adzick et al., who described 134 fetuses with CPAM (36). Of these, 14 pregnancies were terminated, 101 cases were managed expectantly, 13 women underwent open fetal surgery, and 6 fetuses underwent thoracoamniotic shunt placement. In the fetuses that did not develop nonimmune hydrops, the postnatal survival was 100%. In contrast, of 25 large CPAMs that developed hydrops and were managed expectantly, there was 100% mortality, with death in utero or immediately after birth. Among the 76 fetuses with CPAMs that were not associated with hydrops, the uniform survival was, in part, due to planned near-term delivery at a tertiary care center. Many of the babies with large lesions required substantial ventilatory support, and four needed support with extracorporeal membrane oxygenation (ECMO).
Fifteen CPAM lesions appeared large at 20 to 26 weeks of gestation with an associated contralateral mediastinal shift, but then clearly decreased in size during the third trimester with the return of the position of the heart toward midline. Although four of these shrinking lesions were associated with polyhydramnios, including one case with fetal ascites, these phenomena resolved as the masses decreased in size.
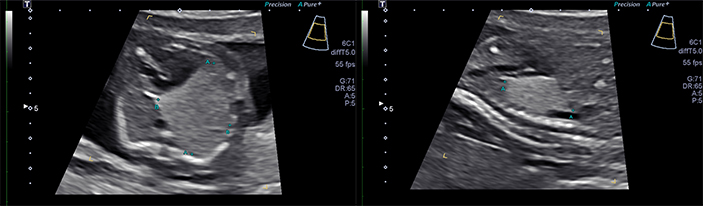
Crombleholme et al., reported the use of CPAM volume and the CPAM volume ratio (CVR) which helped define the prenatal natural history of CPAMs and as a predictor of the development of hydrops (34). The CPAM volume is calculated using the formula for the volume of an ellipse (h × w × l × 0.52 in cm3) with the measurement of the greatest length in the sagittal section and the width and height taken at 90 degrees to the sagittal measurement. The CPAM volume ratio (CVR) is obtained by dividing the CPAM volume by the head circumference (in cm) to correct for any differences in gestational age. Based on 32 fetuses with CPAMs, the CPAM volume and the CVR at presentation were found to be significantly higher in fetuses destined to develop hydrops. The mean CVR plus 2 standard deviations of fetuses that did not develop hydrops yielded a CVR of 1.6 which comprised 95% of CPAMs with a favorable prognosis. Only 2% of fetuses with CVR < 1.6 developed hydrops, and all of these had a dominant cyst. Of those fetuses with CVRs >1.6, 80% developed hydrops. The CVR may therefore be a useful criterion for selecting fetuses at the greatest risk for the development of hydrops and those at low risk for the development of hydrops (34).
The use of the CVR allowed the prenatal natural history of CPAMs to be better defined. CPAMs are rarely diagnosed prior to 20 weeks gestation and then grow rapidly until a mean gestational age of 26 weeks at which time the growth plateaus. After this point, the CVR declines as the baby grows around the CPAM. This behavior is useful in following fetuses with CPAMs as once a growth plateau is reached the CPAM will regress in size.
The initial evaluation of the patient with a suspected fetal CPAM should include a detailed ultrasound examination to confirm the diagnosis, including color flow Doppler studies to demonstrate or exclude systemic blood supply as seen in BPS. The size of the cysts within the lesion should be noted, as well as the size and location of the CPAM. Evidence of mediastinal shift and subtle signs of hydrops should be sought. The incidence of chromosomal anomalies in CPAM is uncertain. In the report by Adzick et al., among 134 prenatally diagnosed CPAMs, there was only one fetus with a chromosomal abnormality (trisomy 21), for an incidence of only 0.7% (35). Amniocentesis for karyotype analysis may be reasonable if fetal treatment is anticipated (84). Fetal echocardiography should be performed in all cases of suspected CPAM because of an increased incidence of associated cardiac anomalies, particularly truncus arteriosus, and tetralogy of Fallot (1,2). In addition, there is impaired cardiac function in large CPAMs that shifts the mediastinum causing compression of the ventricles, elevating central filling pressures, altering ventricular inflow patterns, and causing reversal of IVC flow with atrial contractions. This pattern of restrictive ventricular filling associated with flow reversals in the IVC with atrial contractions may be a harbinger of the development of hydrops (85). Prenatal consultation should be obtained from a pediatric surgeon, a neonatologist, and a pediatric cardiologist.
If there are associated life-threatening congenital anomalies, the family may choose not to continue the pregnancy. The development of the maternal “mirror syndrome” warrants immediate delivery. A fetus with an isolated CPAM, but no hydrops, should be followed closely by serial sonography. After reaching a growth plateau these lesions may regress and are no longer at risk for developing hydrops.
The use of betamethasone in high-risk CPAMs has become standard practice despite the lack of direct evidence that steroids arrest the growth of CPAMs. In a systematic review of the efficacy of antenatal steroids in the management of high-risk CPAMs, Patwardhan et al. identified 13 observational studies involving 163 pregnancies complicated by CPAM that were treated by single or multiple courses, of antenatal steroids (86). They found a significant mean decrease in the CVR of 1.16 and resolution of hydrops in 86%. Not all patients respond to a single course of steroids, and Morris et al., have reported that two-thirds of patients who do not respond to an initial course of steroids will respond to a second course (87). Fetal surgery for CPAMs is now reserved for only those cases that prove refractory to at least two courses of steroids.
The management of CPAM and hydrops depends on the CVR value that is obtained at presentation. If the CVR is less than 1.6 and there is no evidence of a dominant cyst, the CPAM has little risk for the development of hydrops (34). The fetus should have weekly sonograms to measure the CPAM volume and CVR in order to identify early signs of hydrops or, more likely, that the plateau in growth has been reached. Once the growth plateau is reached, the pregnancy is no longer at risk for the development of hydrops. The surveillance of the fetus can be reduced but one should continue to assess the size of the CPAM, the risk of pulmonary hypoplasia, or “air trapping” that would influence delivery management.
If there is a dominant cyst, even if the CVR is less than 1.6, the fetus remains at significant risk for acute enlargement of the cyst and development of hydrops. A thoracoamniotic shunt may be considered in these cases at the very earliest sign of hydrops.
If the CVR is more than 1.6 at presentation, with or without a dominant cyst, there is up to an 80% chance of hydrops developing (35). Twice weekly sonographic surveillance should be started to help detect the earliest signs of hydrops. Maternal administration of antenatal corticosteroids should be considered in all cases with a CVR of 1.6 at presentation. To date, there have been 13 case series reported with apparent resolution of hydrops in patients with CPAMs that were treated with antenatal steroids (87-89). It is thought that steroids may arrest the growth of the solid component of the CPAM inducing an early growth plateau and allowing the fetus to grow around the CPAM and hydrops to resolve. Due to the lack of a prospective randomized clinical trial, it is not proven that steroids truly affect the growth of CPAMs. It is still possible that the observations reported may simply reflect CPAMs naturally entering a growth plateau regardless of steroid administration.
Fetuses with CPAM and a dominant cyst in which hydrops develop prior to 32 weeks can be considered for treatment in utero. There now have been multiple reports of cystic CPAMs successfully treated by thoracoamniotic shunting (37,38,73,75,90-94) with the survival of 70% of fetuses. Thoracoamniotic shunting for CPAMs with a large cystic component is now considered routine. Many of these cysts do not communicate, however, which may limit the reduction in volume that can be achieved with thoracoamniotic shunt alone. Crombleholme and colleagues have used fetal thoracoscopy to fenestrate the cyst walls of a CPAM to enhance the reduction in volume obtained with thoracoamniotic shunting (see Figures 4-7 below). Typically, up to 50% reduction in CPAM volume may be achieved with thoracoamniotic shunt alone. In contrast, fetal thoracoscopic fenestration of CPAM cysts plus thoracoamniotic shunt can achieve up to 70% reduction in CPAM volume. However, these survivors may still be at risk for associated pulmonary hypoplasia and marked respiratory insufficiency at birth and some have required high-frequency ventilation or even ECMO support.
Figure 4. The illustration shows the fetoscope has been inserted into the fetal chest and into the dominant cyst of the CPAM with a laser fiber (green tip) extended at the end of the fetoscope for laser fenestration of non-communicating cysts in the CPAM.
Figure 5. The illustrations shows a close up of the laser fiber extended from the tip of the fetoscope in order to create a communication with the dominant cyst of non-communicating cyst by using laser energy to burn a hole in the cyst wall.
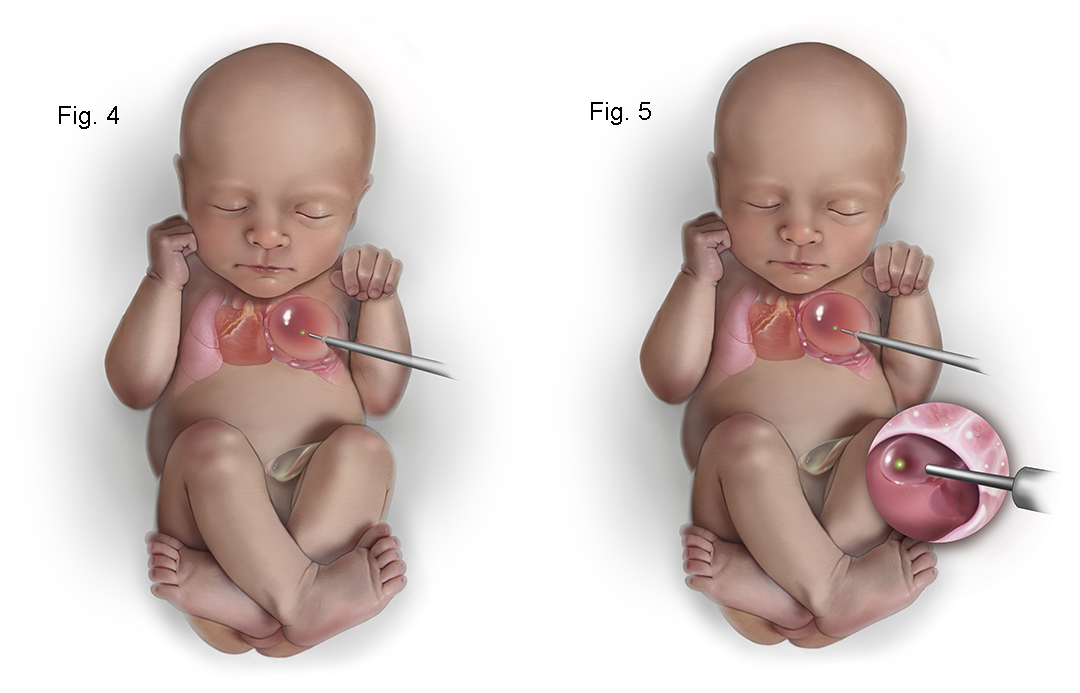
Figure 6. The illustration shows a close up view of the internal CPAM showing communication of the cysts with the dominant cyst allowing more complete decompression of the CPAM.
Figure 7. The illustration shows the placement of a thoracoamniotic shunt with a double pigtail catheter which allows drainage of fluid within the CPAM to the amniotic cavity. Internally the shunt sits with a pigtail within the CPAM and it passes through the chest wall with a pigtail on the outside to drain fluid into the amniotic cavity.
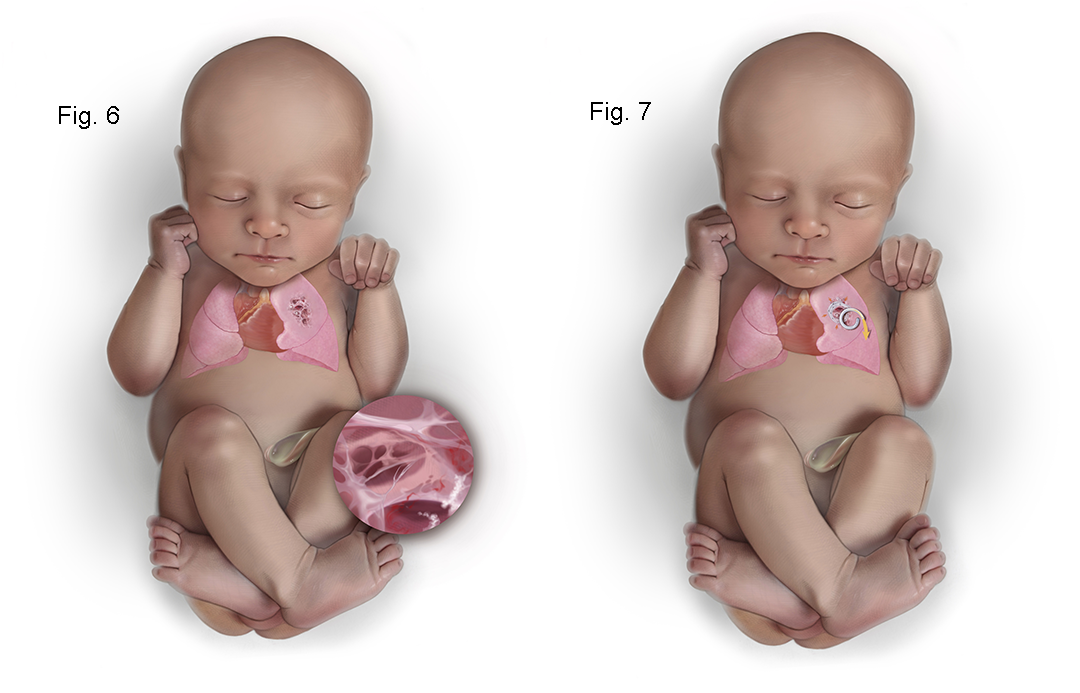
The most challenging antenatal presentation is large microcystic CPAM with hydrops that does not lend itself to shunt decompression. Fetal surgical resection of massively enlarged microcystic CPAM with associated hydrops has been performed in at least 25 patients at 21 to 29 weeks gestation (58,95). In this series, of the 16 fetuses that survived (64%), CPAM resection led to hydrops resolution in 1 to 2 weeks, return of the mediastinum to the midline within 3 weeks, and impressive in utero lung growth. There were also nine fetal deaths in this series.
The need for open fetal surgery for CPAM has largely disappeared since the advent of steroid use to arrest the growth of these lesions. Open fetal surgery presents significant potential risks for the mother with only ~64% survival for affected fetuses who undergo the procedure. An attractive alternative that is far less invasive than open fetal surgery is ultrasound-guided intravascular laser ablation of the lobar branch of the pulmonary artery supplying the CPAM (96) (see Figures 8-9 below). The CPAM is not functional lung tissue and there is little downside to ablation of the branch pulmonary artery to the CPAM. There is limited experience with treating CPAMs with this approach but more experience in treating BPS (97). However, this approach to devascularize the CPAM appears to be safe involving much less risk for the mother with good expectation for survival of the fetus.
Figure 8. The illustration shows how a type III hybrid CPAM can be treated with intravascular laser photocoagulation. An 18 gauge needle is passed under ultrasound into the CPAM into the systemic feeding vessel and the laser fiber is inserted into the vessel just beyond the tip of the 18 gauge needle. Turing on the laser fiber uses light to coagulate the systemic feeding vessel which prevents the CPAM from growing and causes it to regress in size.
Figure 9. The illustration shows the 18 gauge needle in the systemic feeding vessel with the laser fiber extended into the vessel lumen about to be fired to photocoagulate the vessel.
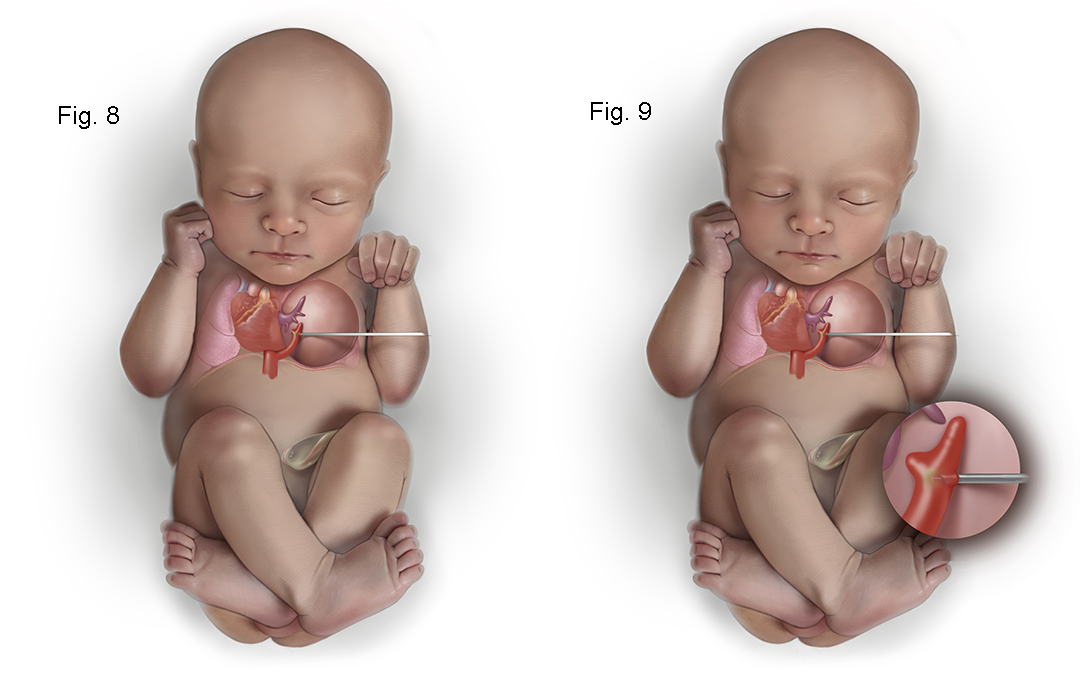
As mentioned above, a course of maternal steroids (betamethasone or dexamethasone) may be effective in arresting the growth of the CPAM (86-89). In some instances, the size of the CPAM remains substantial with significant mediastinal shift and cardiac compression. In these cases, delivery by EXIT-to-Resection may be indicated (98). The rationale for this approach is that the mediastinal shift and compression by the CPAM will make ventilation difficult and will similarly impair venous return if ECMO is attempted. During EXIT-to-Resection, a thoracostomy for resection of the CPAM is performed on placental support. In this approach when the infant is born the trachea is decompressed facilitating ventilation, and if ECMO is needed, venous return will be unobstructed (98). In a small series of 9 cases of large CPAMs managed by EXIT-to-Resection, Hedrick et al reported 8 of 9 survived and 4 subsequently required ECMO support (98).
In determining the best setting for the delivery of a fetus with a CPAM, prenatal prognostic variables can be very helpful. In cases in which the CVR is < 1.6 at presentation, it is rare for these CPAMs to grow to a size that would compromise the fetus. In these cases, delivery close to home should be planned with postnatal evaluation of asymptomatic cases at 2-3 months of age. In contrast, CPAMs that have a CVR of >1.6, a LMVR > 2.0, or have a large cystic component are at greater risk of pulmonary hypoplasia and are more likely to be symptomatic at birth. In these cases, delivery in a tertiary care setting with Neonatologists and Pediatric Surgeons immediately available is advisable. Those CPAMs with large cystic components are a subset at risk for “air trapping” after delivery. Although CPAMs do not have direct communication with the tracheobronchial tree, the cysts within the CPAM rapidly absorb fluid following delivery but do not readily absorb air and in some cases will progressively enlarge shifting the mediastinum and compromise venous return to the heart (1,99). This “air trapping” may occur within hours of delivery especially if the infant requires positive pressure ventilation due to associated pulmonary hypoplasia. Air trapping in this setting may be life-threatening if not dealt with expeditiously by emergency thoracotomy and resection or by cannulation for ECMO support to facilitate stability for resection. These cases should be delivered in a tertiary care setting where appropriate Neonatal, Pediatric Surgery, Pediatric Anesthesia, and ECMO capability are all immediately available. In cases with severe mediastinal shift, selective intubation of the contralateral bronchus may be a useful temporizing measure until resection of the CPAM can be accomplished. Pneumothorax is an additional concern in CPAM, especially in the type I or II or IV lesions, and may require tube thoracostomy (100).
In general, the diagnosis of CPAM does not affect the decision-making regarding the mode of delivery except in one specific instance. Most cases of CPAM should be able to deliver vaginally with a cesarean section reserved for Obstetric indications. CPAMs associated with intrathoracic airway compromise due to significant shift of the mediastinal structures and complete effacement of the intra-thoracic airway may preclude normal oxygenation and ventilation. While the Neonatologist will have no trouble intubating the infant, the more distal obstruction of the airway may result in significant hypercapnia and hypoxemia. In this instance, only ECMO support may stabilize the baby prior to resection of the CPAM. In order to avoid an emergency ECMO cannulation, in babies identified with intra-thoracic airway compromise, an EXIT-to-Resection strategy will allow restoration of the airway prior to delivery converting an emergent situation to a more controlled one.
There is no data to suggest that early delivery offers any benefit to a baby with a CPAM. An argument can be made for a planned delivery, either by induction of labor or repeat cesarean section slightly early at 38-39 weeks gestation. The main advantage here is in the cases in which it is anticipated the baby will be symptomatic at birth, either due to pulmonary hypoplasia or “air trapping” within the cystic component of the CPAM. In less high-risk cases, waiting for the mother to go into labor is appropriate.
In all CPAM cases, there should be conscious decision-making about the site, mode, and timing of delivery. The delivery plan should take into account the nature of the CPAM, whether there is evidence of intra-thoracic airway compromise, cystic components at risk for “air trapping”, CVR or LMVR suggesting an increased risk of pulmonary hypoplasia and the resources available to support the baby at the delivery hospital. In the majority of CPAMs, there is no reason to change the planned delivery at the patient’s local hospital because the CPAM will be asymptomatic at birth. We often will re-evaluate the CPAM at ~34 weeks’ gestation before making a final recommendation regarding the delivery plan. A CPAM is confined to a single lobe and confined.
In the majority of CPAM patients, there is nothing different about the Neonatal assessment and management of the baby. Most low-risk CPAMs babies will be asymptomatic at birth and the prenatal knowledge of the CPAM allows the Neonatologist to be alert to subtle signs such as perioral cyanosis, acrocyanosis, or persistent tachypnea, which should prompt further investigation.
In high-risk CPAMs, which do not have intra-thoracic airway compromise, the Neonatologist should look for signs of respiratory distress, hypoxia, and pulmonary hypertension, which may be progressive during the resuscitation. The need for intubation and mechanical ventilator support should raise suspicion of a more significant CPAM with associated pulmonary hypoplasia and pulmonary hypertension. A stat portable chest radiograph and echocardiogram should be obtained to better assess the severity of the situation and guide management. A Pediatric Surgeon should be urgently consulted in case an emergency thoracotomy is necessary. Even if emergency resection is not necessary, a symptomatic CPAM should prompt a thoracotomy and resection as soon as practicable as all symptomatic CPAMs require surgical removal.
It is rare that the prognosis in CPAM is so poor that comfort care may be the most appropriate choice for management. In some cases, however, significant associated anomalies may take priority in a decision to offer only comfort care. Even in severe cases of CPAM in which EXIT-to-Resection may be planned, Perinatal Palliative Care consultation may provide needed support for the families during the stressful postnatal course. In many centers, Perinatal Palliative Care consultation is routine for a baby diagnosed with a significant anomaly even if not life-threatening.
Many cases of microcystic CPAM were thought to “disappear” during the 3rd trimester at 32 to 34 weeks gestation. But the sonographic appearance if the lung changes with advancing gestational age. In lungs with a CPAM, the adjacent normal lung becomes isoechogenic with the CPAM at ~32-34 weeks, rendering it sonographically invisible. As fetal MRI has demonstrated, these CPAMs may sonographically “disappear” but are nonetheless still present. The asymptomatic low-risk CPAM does not require a plane radiograph following delivery. These small lesions are frequently hard or impossible to see on plain chest X-rays. Exceptions to this are CPAMs in which there is a significant cystic component at risk for “air trapping.” In these cases, a chest X-ray shortly after delivery is advisable as a baseline with serial follow-up chest films to monitor for progressive “air trapping” potentially causing mediastinal shift and respiratory compromise.
Symptomatic CPAMs should have chest X-rays shortly after delivery to assess the patency of the intra-thoracic airway, mediastinal shift, and compression of normal lung, plus rule out evidence of “air trapping” in cystic components and pneumothorax. Depending on how symptomatic the baby is, further evaluation prior to surgery. If there is early evidence of “air-trapping,” an emergency thoracotomy for resection of the CPAM is advisable as this situation will only worsen with time. If unstable, consideration should be given to intubation of the contralateral mainstem bronchus and cannulation for ECMO support before proceeding with thoracotomy and resection on ECMO. If there is a dominant cyst that has overinflated causing marked mediastinal shift and hemodynamic compromise, placing a chest tube into the cyst may temporize the situation until the thoracotomy and resection can be performed.
In over 75% of cases, CPAMs are asymptomatic and the timing of cross-sectional imaging, either MRI or CT scan with IV contrast, will depend on characteristics that suggest risk for complications. In newborns at increased risk for complications, even if asymptomatic, include bilateral CPAMs, multifocal macrocysts, associated pneumothorax, or family history of pleuropulmonary blastoma-associated conditions (renal cystic disease, small bowel polyps, childhood cancers) or in type I or type IV CPAMs which cannot be radiographically distinguished from congenital cystic pleuropulmonary blastoma (101-103). In type I or type IV CPAMs, CPAMs associated with pneumothorax, or with a family history suggesting an increased risk of pleuropulmonary blastoma, we recommend resection shortly after delivery. The reason for this recommendation is that pleuropulmonary blastoma follows a predictable pattern of histologic evolution with lesions left in situ longer transitioning from a predominantly cystic to a more solid lesion with a worse prognosis. In congenital cystic pleuropulmonary blastoma surgical resection is usually curative but as the tumor becomes more solid, it becomes less likely to be cured by surgery alone and is often resistant to adjunctive chemotherapy and/or radiation therapy.
In the absence of symptoms or factors associated with pleuropulmonary blastoma, we routinely defer obtaining a thin-section CT scan with IV contrast until the baby is sufficiently old enough to have an outpatient CT scan with sedation without requiring admission for apnea monitoring. In a term infant, this is more than 45 post-conceptual weeks, while in the preterm infant (<36 weeks), this is usually after 55 post-conceptual weeks of age.
Some have suggested that prenatal imaging with MRI may reduce the need for postnatal imaging. We find postnatal imaging by thin-section CT scan with IV contrast to be helpful in planning the operative approach, determining the ability to perform lung-sparing resections, and avoiding surgery altogether for cases thought to be type III CPAMs, which prove to be due to segmental or lobar hyperinflation on postnatal imaging. The interval between fetal MRI and the postnatal CT scan may be up to 20 weeks or more. During this interval, there may have been significant regression of the CPAM and/or growth of the normal lung around the CPAM. Postnatal thin section high-resolution CT scans (1.5-3 mm sections) with IV contrast can confirm the diagnosis, demonstrate systemic feeding vessels, if present, and allow determination of whether lobectomy or segmental resection will be possible. This is not just related to the size of the CPAM, but to its location within the lobe. Even a relatively small CPAM, that is located in the hilum of the lobe, may not be amendable to anything less than a formal lobectomy, due to a compromise of vascular supply or bronchial connection to the more peripheral normal lung parenchyma in the lobe. Conversely, even moderately large CPAMs, which are peripheral in location, may be amendable to lung-sparing resection.
Postnatal thin-section high-resolution CT scans with IV contrast may also be better able to distinguish homogeneous type III CPAMs from hyperinflation of segments or lobes than fetal MRI. This may not be possible on prenatal MRI, but the normal vascular distribution with emphysematous lung parenchyma is usually clear on CT scans with contrast, which obviates the need for resection. These hyperinflated lobes or segments are thought to be due to the trapping of air (or lung fluid prenatally) due to abnormally soft bronchi which usually resolves during the first year of the life and rarely causes symptoms. The lung tissue itself is histologically normal and resection should be avoided except in cases of recurrent infection or progressive mass effect on adjacent normal lungs.
There is no medical management for CPAM. Controversy persists as to whether all CPAMs should be resected or observed until complications arise (104). One problem with observation alone is that no one has determined how these lesions could or should be followed for 80 years or more, or how to anticipate complications before they occur. The risks of resection and technical difficulty increase significantly once complications have occurred, potentially compromising outcomes (104).
CPAM is usually confined to a single lobe. Rare cases have been reported of multilobar involvement of one lung or bilateral lesions (105). Complete resection of the CPAM, by lobectomy or segmental resection, is the treatment of choice. In cases of extensive involvement of nearly the entire lung, resection of multiple lobes or pneumonectomy may rarely be necessary. There are several reports, however, detailing potentially lethal problems associated with pneumonectomy in newborns resulting from mediastinal shift with vascular compression of the trachea and remaining bronchus (106). Because of these risks, some groups advocate a nonanatomic resection to preserve as much pulmonary parenchyma as possible to allow postoperative compensatory growth and avoid postpneumonectomy complications (107).
The newborn with a CPAM detected antenatally that subsequently regresses, also needs careful postnatal evaluation (36,83). Often subtle abnormalities will be evident on chest radiography, but chest CT or MRI scanning may be necessary to detect residual CPAM tissue. Several authors have recommended that as long as these lesions are asymptomatic, they be observed closely and managed without resection (58,83,108,109). The arguments against this approach include the reported cases of myxosarcoma, embryonal rhabdomyosarcoma, pleuropulmonary blastoma, and bronchoalveolar carcinoma arising in CPAMs (110-115). While primary lung tumors are rare during the first two decades of life, 4% of those reported were associated with congenital cystic lesions of the lung, including CPAM (116). While CPAM-associated malignancies often arise only after decades, the youngest patient reported with malignancy was only 13 months of age (Ozcan et al., 2001). Because there is an anomalous connection to the tracheobronchial tree, infection is an additional potential complication (110,112,113,116-135). Some have argued that asymptomatic CPAMs should be followed expectantly, and that the risks of surgery in infancy outweigh the potential benefits (108). Once a CPAM has become infected, the incidence of postoperative complications increases from 10 to 32% (104). However, the continued presence of CPAM represents a life-long risk of both infection and malignant transformation. In centers with significant experience in lung resection in infants, CPAMs can be safely resected with no mortality and virtually no morbidity (52,136). Our approach is to obtain a postnatal CT scan and perform a muscle-sparing thoracotomy for lobectomy or nonanatomic resection whenever possible to retain normal lung tissue. An added benefit to resection over observation is that the remaining lung undergoes significant compensatory growth within months of the surgery (52). This does not occur if the CPAM is left in situ.
The rationale for resection of asymptomatic CPAM includes several factors. CPAMs have a circuitous communication with the tracheobronchial tree, which makes them prone to infection, and do not have the normal lung mechanisms of clearing secretions and bacteria. The incidence of infection arising in CPAMs is hard to estimate but some put this figure in the 5-10% of cases (134,135). The second factor favoring resection is the development of malignant tumors within the CPAM. Up to 4% of all malignant lung tumors resected from patients < 20 years of age arose within a CPAM. The most common histologies are bronchoalveolar carcinoma (137) and rhabdosarcoma (138,139). Pleuropulmonary blastoma has been reported to occur in CPAMs, but is far more likely that these were congenital cystic pleuropulmonary blastomas mistakenly thought to be type IV CPAMs. Another reason for resection of CPAMs is to foster compensatory lung growth, which only occurs in response to the removal of the CPAM. In cases of hybrid CPAMs with a systemic feeding vessel, there is the risk of high-output cardiac failure from blood flow through the CPAM, although this appears to be more common in bronchopulmonary sequestration. In addition, hemorrhage into the CPAM from an anomalous systemic feeding vessel may occur.
There are two forms of minimally invasive surgical approaches to the resection of CPAMs: thoracoscopic resection and muscle-sparing thoracotomy. There has not been a head-to-head comparison between these approaches, but there is general consensus that a traditional posteriolateral thoracotomy with division of the latissimus dorsi and anterior serratus muscles should be avoided as it results in decreased shoulder mobility and may predispose to scoliosis. More important to the outcome is the experience of the operating surgeon. In order to perform what is essentially a prophylactic operation in asymptomatic cases, a surgeon should have significant experience with essentially no mortality and very low morbidity to justify the surgery.
In prenatally diagnosed CPAMs, the overall survival rate is over 95% with much of the mortality associated with the subset that develops non-immune hydrops. In most symptomatic (and virtually all asymptomatic) CPAMs, resection is curative and the prognosis is excellent (140-143). Long-term follow-up of these patients has been limited. In asymptomatic CPAMs that underwent resection prior to one year of age, over 80% had normal pulmonary function testing during childhood (142). In contrast, among symptomatic CPAMs managed surgically, 50% had mildly abnormal PFTs and mild exercise intolerance by 8 years of age (145). In a similar cohort managed by surveillance only, PFTs were normal, but there was still mildly reduced exercise tolerance.
The long-term prognosis is thought to vary with the type of CPAM with type I lesions having the best prognosis, and types II and III somewhat less favorable, which may relate to associated anomalies in type II lesions and worse associated pulmonary hypoplasia and pulmonary hypertension in type III CPAMs. Type IV CPAMs have equivalent outcomes to type I CPAMs. However, in cases of congenital cystic pleuropulmonary blastoma mistaken for type IV CPAMs, the prognosis tracks with PPB, not CPAM.
The long-term outcome of infants with CPAM following resection is excellent. If residual CPAM is left behind or the mass is not resected, the child will be at risk for infectious and potentially malignant complications. Also, as noted above, infants usually have remarkable compensatory growth of the residual lung following resection, with continued s for up to eight years. We also recommend prophylaxis against respiratory syncytial virus (RSV) in infancy in children with significant pulmonary hyperplasia, pulmonary hypertension, or chronic lung disease. Children who survived open fetal surgery for CPAMs associated with hydrops appear to be still doing well more than 20 years postoperatively.
CPAM has no known genetic defect responsible for its development and is thought to be an early developmental anomaly of uncertain cause. CPAM is not known to be specifically associated with chromosomal abnormalities, although one case of the 134 CCAMs reported by Adzick et al., had trisomy 21 (36). No cases of recurrence of CPAM in a sibling or offspring have been reported.
1. Stocker JT, Madewell JER, Drake RM. Congenital cystic adenomatoid malformation of the lung: classification and morphologic spectrum. Hum Pathol. 1977;8:155-171.
2. Miller RK, Sieber WK, Yunis EJ. Congenital cystic adenomatoid malformation of the lung: a report of 17 cases and review of the literature. Pathol Annu. 1980;1:387-407.
3. Shanji FM, Sachs JH, Perkins DG. Cystic diseases of the lungs. Surg Clin North Am. 1988;68:581-618.
4. Leninger BJ, Haight C. Congenital cystic adenomatoid malformation of the left lobe lower lobe with compression of remaining lung. Clin Pediatr. 1973;12:182-186.
5. Demos NJ, Teresi A. Congenital lung malformations: a unified concept and a case report. J Thorac Cardiovasc Surg. 1975:70:260-264.
6. Cochia R, Sobonya RE. Congenital cystic adenomatoid malformation of the lung and bronchial atresia. Hum Pathol. 1981;12:947-950.
7. Moerman P, Fryns JP, Vandenberghe K, et al. Pathogenesis of congenital cystic adenomatoid malformation of the lung. Histopathology. 1992;21:315-321.
8. Langston C. New concepts in the pathology of congenital lung malformations. Semin Pediatr Surg. 2003;12:17-37.
9. Keswani SG, Crombleholme TM, Pawel BR, et al. Prenatal diagnosis and management of mainstem bronchial atresia. Fetal Diagn Ther. 2005;20:74-78.
10. Kunisaki SM, Fauza DO, Nemes LP, et al. Bronchial atresia: the hidden pathology within a spectrum of prenatally diagnosed lung masses. J Pediatr Surg. 2006;41:61-65.
11. Hernanz-Schulman M. Cysts and cystlike lesions of the lung. Radiol Clin North Am. 1993;31:631-649.
12. Rashad F, Gaisoni E, Gaglione S. Aberrant arterial supply in congenital cystic adenomatoid malformation of the lung. J Pediatr Surg. 1988;23:107-108.
13. Cass DL, Crombleholme TM, Howello LJ, et al. Cystic lung lesions with systemic arterial blood supply: a hybrid of congenital cystic adenomatoid malformation and bronchopulmonary sequestration. J Pediatr Surg. 1997;32:986-990.
14. Stocker JT. Congenital pulmonary airway malformation: a new name and an expanded classification of congenital cystic adenomatoid malformation of the lung. Histopathology. 2002;41:424-431.
15. Davidson LA, Batman P, Fagan DG. Congenital acinar dysplasia: a rare cause of pulmonary hypoplasia. Histopathology. 1998;32:57-59.
16. Priest JR, Williams GM, Hill DA, et al. Pulmonary cysts in early childhood and the risk of malignancy. Pediatr Pulmonol 2009; 44:14.
17. Stocker JT. Cystic lung disease in infants and children. Fetal Pediatr Pathol 2009; 28:155.
18. Walker J, Cudmore RE. Respiratory problems and cystic adenomatoid malformation of the lung. Arch Dis Child. 1990;65:649-659.
19. Adzick NS, Harrison MR, Glick PL, et al. Fetal cystic adenomatoid malformation: prenatal diagnosis and natural history. J Pediatr Surg. 1985b;20:483-488.
20. Harrison MR, Adzick NS, Jennings RW, et al. Antenatal intervention for congenital cystic adenomatoid cystic malformation. Lancet. 1990a;336:965-967.
21. van Koningsbruggen S, Ahrens F, Brockmann M, et al. Congenital cystic adenomatoid malformation type 4. Pediatr Pulmonol 2001; 32:471.
22. Chowdhury MM, Chakraborty S. Imaging of congenital lung malformations. Semin Pediatr Surg 2015; 24:168.
23. MacSweeney F, Papagiannopoulos K, Goldstraw P, Sheppard MN, Corrin B, Nicholson AG. An assessment of the expanded classification of congenital cystic adenomatoid malformations and their relationship to malignant transformation. Am J Surg Pathol. 2003;27(8): 1139-1146.
24. Hill DA, Dehner LP. A cautionary note about congenital cystic adenomatoid malformation (CCAM) type 4. Am J Surg Pathol. 2004;28(4): 554-555.
25. Adzick NS, Harrison MR, Glick PL, et al. Diaphramatic hernia in the fetus: prenatal diagnosis and outcome in 94 cases. J Pediatr Surg 1985a 20: 357-362
26. Hobbins JC, Grannum PAT, Berkowitz RL, et al. Ultrasound in the diagnosis of congenital anomalies. Am J Obstet Gynecol. 1979;134: 331-345.
27. Gonzalez-Cuezzi F, Boggs JD, Raffensberger JG. Brain heteropia in the lungs: a rare case of respirator disease in the newborn. Am J Clin Pathol. 1980;73:281-285.
28. Romero R, Chernenak FA, Katzen J, et al. Antenatal sonographic findings of extralobar pulmonary sequestration. J Ultrasound Med. 1982;1: 131-132.
29. Chinn DH, Filly RA, Callen PW, et al. Congenital diaphragmatic hernia diagnosed prenatally by ultrasound. Radiology. 1983;148:119-123.
30. May DA, Barth RA, Yeager S, et al. Perinatal and postnatal chest sonography. Radiol Clin North Am. 1993;31:499-516.
31. Hubbard AM, Crombleholme TM. Prenatal and neonatal lung lesions. Semin Roentgenol. 1998;33:117-125.
32. Carter R. Pulmonary sequestration. Ann Thorac Surg. 1959;7:68-88.
33. Hernanz-Schulman M, Stein IM, Neblett WW, et al. Pulmonary sequestration: diagnosis with color flow sonography and a new theory of associated hydrothorax. Radiology. 1991;180:817-821.
34. Morin L, Crombleholme TM, Lewis F, et al. Bronchopulmonary sequestration: prenatal diagnosis with clinicopathologic correlation. Curr Opin Obstet Gynecol. 1994;6:479-481.
35. Crombleholme TM, Leichtly KW, Howell LJ, et al. Cystic adenomatoid malformation volume ratio predicts outcome in prenatally diagnosed cystic adenomatoid malformation of the lung. J Pediatr Surg. 2002;37:331-338.
36. Adzick NS, Harrison MR, Crombleholme TM. Fetal lung lesions: management and outcome. Am J Obstet Gynecol. 1998;179:884-889.
37. Nicolaides KH, Blatt AJ, Greenough A. Chronic drainage of fetal pulmonary cysts. Lancet. 1987;1:618-619.
38. Laberge JM, Flageole H, Pugash D, et al. Outcome of the prenatally diagnosed congenital cystic adenomatoid lung malformation: a Canadian experience. Fetal Diagn Ther. 2001;16:178-186.
39. Gornall AS, Budd JL, Draper ES, et al. Congenital cystic adenomatoid malformation: accuracy of prenatal diagnosis, prevalence and outcome in a general population. Prenat Diagn 2003; 23:997.
40. Dann SM, Martin JN, White SJ. Antenatal ultrasound findings in cystic adenomatoid malformation. Pediatr Radiol. 1981;10:180-182.
41. Bezzuti RT, Isler RJ. Antenatal ultrasound detection of cystic adenomatoid malformation of lung: report of a case and review of the recent literature. Clin Ultrasound. 1983;11:342-346.
42. Diwan RV, Brennan JN, Phillipson EH, et al. Ultrasonic prenatal diagnosis of Type III congenital cystic adenomatoid malformation of lung. J Clin Ultrasound. 1983;11:218-221.
43. Cone APD, Adam AE. Cystic adenomatoid malformation of the lung (Stocker type III) found on antenatal ultrasound examination. Br J Radiol. 1984;57:176-178.
44. Johnson JA, Rumack CM, Johnson ML, et al. Cystic adenomatoid malformation: antenatal demonstration. AJR Am J Roentgenol. 1984; 142:483-484.
45. Morcos SF, Lobb MO. The antenatal diagnosis by ultrasonography of type III congenital cystic adenomatoid malformation of the lung: case report. Br J Obstet Gynaecol. 1986;93:1002-1005.
46. Mendoza A, Wolf P, Edwards DK, et al. Prenatal ultrasonographic diagnosis of congenital adenomatoid malformation of the lung. Arch Pathol Lab Med. 1986;110:402-404.
47. Deacon CS, Smart PJ, Rimmer S. The antenatal diagnosis of congenital cystic adenomatoid malformation of the lung. Br J Radiol. 1990;63:968-970.
48. Boulot P, Pages A, Deschamps F, et al. Early prenatal diagnosis of congenital cystic adenomatoid malformation of the lung (Stocker’s type I): a case report. Eur J Obstet Gynecol Reprod Biol. 1991;41:159-162.
49. Hubbard AM, Adzick NS, Crombleholme TM, et al. Congenital chest lesions: diagnosis and characterization with prenatal MR imaging. Radiology 1999; 212:43.
50. Levine D, Barnewolt CE, Mehta TS, et al. Fetal thoracic abnormalities: MR imaging. Radiology 2003; 228:379.
51. Zeidan S, Gorincour G, Potier A, et al. Congenital lung malformation: evaluation of prenatal and postnatal radiological findings. Respirology 2009; 14:1005.
52. Azizkhan RG, Crombleholme TM. Congenital cystic lung disease: contemporary antenatal and postnatal management. Pediatr Surg Int 2008; 24:643.
53. Kline-Fath BM. Is prenatal sonography accurate in identification of Congenital lung lesions? Scientific paper presented at SPR, Boston, MA. April 15, 2010.
54. Hedrick HL, Flake AW, Crombleholme TM, et al. The ex utero intrapartum therapy procedure for high-risk fetal lung lesions. J Pediatr Surg 2005; 40:1038.
55. Zamora IJ, Sheikh F, Cassady CI, et al. Fetal MRI lung volumes are predictive of perinatal outcomes in fetuses with congenital lung masses. J Pediatr Surg 2014; 49:853.
56. Schott S, Mackensen-Haen S, Wallwiener M, et al. Cystic adenomatoid malformation of the lung causing hydrops fetalis: case report and review of the literature. Arch Gynecol Obstet 2009; 280:293.
57. Stocker, T, Dehner, LP. Pediatric Pathology, 2nd ed, Lippincott Williams and Wilkins, p.473.
58. Adzick NS. Fetal thoracic lesions. Semin Pediatr Surg. 1993;2:103-108. Adzick NS, Harrison MR. Management of the fetus with a cystic adenomatoid malformation. World J Surg. 1993;17:342-349.
59. Atkinson JB, Ford EG, Ketagawa H, et al. Persistent pulmonary hypertension complicating cystic adenomatoid malformations in a neonate. J Pediatr Surg. 1972;27:54-56.
60. Nishibayashi SW, Andrassy RJ, Woolley MM, et al. Congenital cystic adenomatoid malformation: a 30 year experience. J Pediatr Surg. 1981; 16:704-706.
61. Pulpeiro JR, Lopez I, Satelo T, et al. Congenital cystic adenomatoid malformation of the lung in a young adult. Br J Radiol. 1987;60:1128-1132.
62. Heij HA, Ekkelkamp S, Vos A. Diagnosis of congenital cystic adenomatoid malformation of the lung in newborn infants and children. Thorax. 1990;45:122-125.
63. Neilson IR, Russo P, Faberge JM, et al. Congenital adenomatoid malformation of the lung: current management and prognosis. J Pediatr Surg. 1991;26:975-981.
64. Kuller JA, Yankowitz J, Goldberg JD, et al. Outcome of antenatally diagnosed cystic adenomatoid malformations. Am J Obstet Gynecol. 1992;167:1038-1041.
65. Cloutier MM, Schaeffer DA, Hight D. Congenital cystic adenomatoid malformation. Chest. 1993;103:761-764.
66. Adzick NS, Harrison MR, Flake AW, et al. Fetal surgery for cystic adenomatoid malformations of the lung. J Pediatr Surg. 1993;28;806-812.
67. Golladay ES, Mollitt PL. Surgically correctable fetal hydrops. J Pediatr Surg. 1984;19:59-62.
68. Graham D, Winn K, Dex W, et al. Prenatal diagnosis of cystic adenomatoid malformation of the lung. J Ultrasound Med. 1982;1:9-12.
69. Glaves J, Baker JL. Spontaneous resolution of maternal hydramnios in congenital cystic adenomatoid malformation of the lung. Antenatal ultrasound features: case report. Br J Obstet Gynaecol. 1983;90:1065-1068.
70. Heydanus R, Stewart PA, Wladimiroff JW, et al. Prenatal diagnosis of congenital cystic adenomatoid lung malformation: a report of seven cases. Prenat Diagn. 1993;13:65-71.
71. Meagher SE, Fisk NM, Harvey JG, et al. Disappearing lung echogenicity in fetal bronchopulmonary malformations: a reassuring sign? Prenat Diagn. 1993;13:495-501.
72. Etches PC, Tierney AJ, Demianczuk NN. Successful outcome in a case of cystic adenomatoid malformation of the lung complicated by fetal hydrops, using extracorporeal membrane oxygenation. Fetal Diagn Ther. 1994;9:88-91.
73. Dommergues M, Louis-Sylvestre C, Mandelbrot L, et al. Congenital adenomatoid malformation of the lung: when is active fetal therapy indicated? Am J Obstet Gynecol. 1997;177:953-958.
74. Higby K, Melendez BA, Heiman HS. Spontaneous resolution of nonimmune hydrops in a fetus with a cystic anenomatoid malformation. J Perinatol. 1998;18:308-310.
75. Bunduki V, Ruano R, da Silva MM, et al. Prognostic factors associated with congenital cystic adenomatoid malformation of the lung. Prenat Diagn. 2000;20:459-464.
76. Diamond IR, Wales PW, Smith A. Survival after CCAM associated with ascites: a report of a case and review of the literature. J Pediatr Surg. 2003;38:E1-E3.
77. Murayama K, Jimbo T, Masumoto Y, et al. Prenatal diagnosis and management of some fetal intrathoracic abnormalities. Am J Obstet Gynecol. 1987;157;1252-1255.
78. Creasy R. Mirror syndromes. In: Goodlin RC, ed. Care of the Fetus. New York: Masson; 1979:48-50.
79. Roberts JM, Taylor RN, Musci JJ, et al. Preeclampsia: an endothelial cell disorder. Am J Obstet Gynecol. 1989;161:1200-1204.
80. Fine C, Adzick NS, Doubilet PM. Decreasing size of a congenital cystic adenomatoid malformation in utero: case report. J Ultrasound Med. 1988;7:405-408.
81. Saltzman DH, Adzick NS, Benacerraf BR. Fetal cystic adenomatoid malformation of the lung: apparent improvement in utero. Obstet Gynecol. 1988;71:1000-1003.
82. Budorick NE, Pretorius DH, Leopold SR, et al. Spontaneous improvement of intrathoracic masses diagnosed in utero. J Ultrasound Med. 1992;11:653-662.
83. MacGillivray TE, Adzick NS, Harrison MR, et al. Disappearing fetal lung lesions. J Pediatr Surg. 1993;28:1321-1325.
84. D’Alton ME, DeCherney AH. Prenatal diagnosis. N Engl J Med. 1993; 328;114-120.
85. Mahle WT, Rychik J, Tian ZY, et al. Echocardiographic evaluation of the fetus with congenital cystic adenomatoid malformation. Ultrasound Obstet Gynecol. 2000;16(7):620-624.
86. Patwardhin S, Chang E, Sullivan S. Systematic review of the efficacy of antenatal steroids in the management of high-risk microcystic CPAM. Am J Obstet Gynecol 2016; S372-373
87. Morris LM, Lim FY, Livingston JC, Polzin WJ, Crombleholme TM. High-risk congenital pulmonary airway malformations have a variable response to steroids. J Pediatr Surg. 2009;44:60-64.
88. Tsao K, Hawgood S, Vu L, et al. Resolution of hydrops fetalis in congenital cystic adenomatoid malformation after prenatal steroid therapy. J Pediatr Surg. 2003;38(3):508-510.
89. Peranteau WH, Wilson RD, Liechty KW, et al. The effect of maternal betamethasone administration on prenatal congenital cystic adenomatoid malformation growth and fetal survival. Fetal Diagn Ther. 2007;22(5):365-371.
90. Clark SL, Vitale DJ, Minton SC, et al. Successful fetal therapy for cystic adenomatoid malformation associated with second trimester hydrops. Am J Obstet Gynecol. 1987;157:294-297.
91. Bernaschek G, Deutinger J, Hansmann M, et al. Feto-amniotic shunting—report of the experience of four European centres. Prenat Diagn. 1994;14:821-833.
92. Miller JA, Corteville JE, Langer JC. Congenital cystic adenomatoid malformation in the fetus: natural history and predictors of outcome. J Pediatr Surg. 1996;31:805-808.
93. Wilson RD, Baxter JK, Johnson M, et al. Thoracoamniotic shunts: fetal treatment of pleural effusions and congenital cystic adenomatoid malformation. Fetal Diagn Ther. 2004;19:413-420.
94. Viggiano MB, Amaral WNd, Fonseca PPS, et al. Prenatal catheter placement for fetal cystic adenomatoid pulmonary malformation: a case report. Fetal Diagn Ther. 2006;21:194-197.
95. Harrison MR, Adzick NS, Longaker MT, et al. Successful repair in utero of a fetal diaphragmatic hernia after removal of herniated viscera from the left thorax. N Engl J Med. 1990b;322:1582-1584.
96. Ruano R, deA Pimata FJ, Marques DaSilva M et al. Percutaneous intrauterine laser ablation of the abnormal vessel in pulmonary sequestration with hydrops at 29 weeks gestation. J Ultrasound Med 2007; 26: 1235-1241
97. Ruano R, Marques DaSilva M, Assuncao Salutino EM, Kilby MD, Tannuti U, Zagaib M. Percutaneous laser ablation under ultrasound guidance for fetal hyperechogenic microcystic lung masses with hydrops: a single center cohort and literature review. Prenatal Diagn 32(12); 2012:1127-1132
98. Hedrick HL, Flake AW, Crombleholme TM, et al. The ex utero intrapartum therapy procedure for high-risk fetal lung lesions. J Pediatr Surg. 2005;40:1038-1043; discussion 1044.
99. Bailey PV, Tracy T Jr, Connors RH, et al. Congenital bronchopulmonary malformations: diagnostic and therapeutic considerations. J Thorac Cardiovasc Surg. 1990;99:597-603.
100. Bentur L, Canny G, Thoener P, et al. Spontaneous pneumothorax in cystic adenomatoid malformation: unusual clinical and histologic features. Chest. 1991;99:1292-1293.
101. Zhang N, Zeng Q, Ma X, et al. Diagnosis and treatment of pleuropulmonary blastoma in children: A single-center report of 41 cases. J Pediatr Surg 2020; 55:1351.
102. Dusmet M. Adult lung tumours of childhood origin. Semin Pediatr Surg 2015; 24:196.
103. Stewart DR, Best AF, Williams GM, et al. Neoplasm Risk Among Individuals With a Pathogenic Germline Variant in DICER1. J Clin Oncol 2019; 37:668.
104. Kapralik J, Wayne C, Chan E, Nasr A. Surgical versus conservative management of congenital pulmonary airway malformation in children: A systematic review and meta-analysis. J Pediatr Surg 2016; 51:508.
105. Rempen A, Feige A, Wiinsch P. Prenatal diagnosis of bilateral cystic adenomatoid malformation of the lung. J Clin Ultrasound. 1987;15: 3-8.
106. Szarnicki R, Maurseth K, deLoval M, et al. Tracheal compression by the aortic arch following right pneumonectomy. Ann Thorac Surg. 1978; 25:321-324.
107. Mentzer SJ, Filler RM, Phillips J. Limited pulmonary resections for congenital cystic adenomatoid malformation of the lung. J Pediatr Surg. 1992;27:1410-1413.
108. Aziz D, Langer JC, Tuuha SE, et al. Perinatally diagnosed asymptomatic congenital cystic adenomatoid malformation: to resect or not? J Pediatr Surg. 2004;39(3):329-334.
109. Hsieh CC, Chao AS, Chang YL, et al. Outcome of congenital cystic adenomatoid malformation of the lung after antenatal diagnosis. Int J Gynaecol Obstet. 2005;89:99-102.
110. Stephanopoulos C, Catsaros H. Myxosarcoma complicating a cystic hamartoma. Thorax. 1963;18:144-145.
111. Wecla K, Grippo R, Unger R, et al. Rhabdomyosarcoma of lung arising in a congenital cystic adenomatoid malformation. Cancer. 1977;40:383-388.
112. Benjamin DR, Cahill JL. Bronchioloalveolar carcinoma of the lung and congenital cystic adenomatoid malformation. Am J Clin Pathol. 1991;95:889-892.
113. D’Agostino S, Bonoldi F, Derote S, et al. Embryonal rhabdomyosarcoma of the lung arising in cystic adenomatoid malformation: case report and review of the literature. J Pediatr Surg. 1997;32:1381-1383.
114. Casagrande A, Pederiva F. Association between Congenital Lung Malformations and Lung Tumors in Children and Adults: A Systematic Review. J Thorac Oncol 2016; 11:1837.
115. Pai S, Eng HL, Lee SY, et al. Correction: Pleuropulmonary blastoma, not rhabdomyosarcoma in a congenital lung cyst. Pediatr Blood Cancer 2007; 48:370.
116. Ozcan C, Celik A, Ural Z, et al. Primary pulmonary rhabdomyosarcoma arising within cystic adenomatoid malformation: a case report and review of the literature. J Pediatr Surg. 2001;36:1062-1065.
117. Ueda K, Gruppo R, Unger F, et al. Rhabdomyosarcoma of lung arising in congenital cystic adenomatoid malformation. Cancer. 1977;40:383-388.
118. Weinberg AG, Durrarino G, Moore GC, et al. Mesenchymal neoplasia and congenital pulmonary cysts. Pediatr Radiol. 1980; 9: 179-182
119. Krous HF, Sexauer CL. Embryonal rhabdomyosarcoma arising within a congenital bronchogenic cyst in a child. J Pediatr Surg. 1981;16:506-508.
120. Weinblatt ME, Siegel SE, Isaacs H. Pulmonary blastoma associated with cystic lung disease. Cancer. 1982;49:669-671.
121. Prichard MG, Brown PJ, Sterret GF. Bronchioloalveolar carcinoma arising in longstanding lung cysts. Thorax. 1984;39:545-549.
122. Sheffield EA, Addis BJ, Corrin B, et al. Epithelial hyperplasia and malignant change in congenital lung cysts. J Clin Pathol. 1987;40:612-614.
123. Hedlund GL, Bisset GS III, Bove KE. Malignant neoplasms arising in cystic hamartomas of the lung in childhood. Radiology. 1989;173:77-79.
124. Domizio P, Liesner RJ, Dicks-Mireaux C, et al. Malignant mesenchymoma associated with a congenital lung cyst in a child: case report and review of the literature. Pediatr Pathol. 1990;10:785-797.
125. Morresi A, Wockel W, Karg O. Adenomatoid cystic lung abnormality in adults with associated bronchioloalveolar carcinoma. Pathologe. 1995;16:292-298.
126. Ribet ME, Copin MC, Soots JG, et al. Bronchioloalveolar carcinoma and congenital cystic adenomatoid malformation. Ann Thorac Surg. 1995;60:1126-1128.
127. Kaslovsky RA, Purdy S, Dangman BC, et al. Bronchioloalveolar carcinoma in a child with congenital cystic adenomatoid malformation. Chest. 1997;112:548-551.
128. Granata C, Gambini C, Balducci T, et al. Bronchioloalveolar carcinoma arising in congenital cystic adenomatoid malformation in a child: a case report and review on malignancies originating in congenital cystic adenomatoid malformation. Pediatr Pulmonol. 1998; 25:62-66.
129. De Perrot M, Pache JC, Spiliopoulos A. Carcinoma arising in congenital lung cysts. Thorac Cardiovasc Surg. 2001;49:184-185.
130. Federici S, Comenichelli V, Tani G, et al. Pleuropulmonary blastoma in congenital cystic adenomatoid malformation: report of a case. Eur J Pediatr Surg. 2001;11:196-199.
131. Hasiotou M, Polyviou P, Strantzia CM, et al. Pleuropulmonary blastoma in the area of a previously diagnosed congenital lung cyst: report of two cases. Acta Radiol. 2004;45:289-292.
132. Doladzas T, Arvelakis A, Karavokyros IG, et al. Primary rhabdomyosarcoma of the lung arising over cystic pulmonary adenomatoid malformation. Pediatr Hematol Oncol. 2005;22:525-529.
133. Pai S, Eng HL, Lee SY, et al. Rhabdomyosarcoma arising within congenital cystic adenomatoid malformation. Pediatr Blood Cancer. 2005; 45:841-845.
134. Cook J, Chitty LS, De Coppi P, et al. The natural history of prenatally diagnosed congenital cystic lung lesions: long-term follow-up of 119 cases. Arch Dis Child 2017; 102:798.
135. Thompson AJ, Sidebotham EL, Chetcuti PAJ, Crabbe DCG. Prenatally diagnosed congenital lung malformations-A long-term outcome study. Pediatr Pulmonol 2018; 53:1442.
136. Tsai A, Liechty KW, Hedirsh HL, et al. Outcomes following resection of prenatally diagnosed asymptomatic cystic adenomatoid malformation of the lung. J Pediatr Surg. 2008;43(3):513-517.
137. Casagrande A, Pederiva F. Association between Congenital Lung Malformations and Lung Tumors in Children and Adults: A Systematic Review. J Thorac Oncol 2016; 11:1837.
138. Granata C, Gambini C, Balducci T, et al. Bronchioloalveolar carcinoma arising in congenital cystic adenomatoid malformation in a child: a case report and review on malignancies originating in congenital cystic adenomatoid malformation. Pediatr Pulmonol 1998; 25:62.
139. Ozcan C, Celik A, Ural Z, et al. Primary pulmonary rhabdomyosarcoma arising within cystic adenomatoid malformation: a case report and review of the literature. J Pediatr Surg 2001; 36:1062.
140. Baird R, Puligandla PS, Laberge JM. Congenital lung malformations: informing best practice. Semin Pediatr Surg 2014;23:270
141. Wong A, Vieten D, Singh S, et al. Long-term outcome of asymptomatic patients with congenital cystic adenomatoid malformation. Pediatr Surg Int 2009; 25:479.
142. Davenport M, Warne SA, Cacciaguerra S, et al. Current outcome of antenally diagnosed cystic lung disease. J Pediatr Surg 2004; 39:549.
143. Laje P, Liechty KW. Postnatal management and outcome of prenatally diagnosed lung lesions. Prenat Diagn 2008; 28:612.
144. Tocchioni F, Lombardi E, Ghionzoli M, et al. Long-term lung function in children following lobectomy for congenital lung malformation. J Pediatr Surg 2017; 52:1891.
145. Hijkoop A, van Schoonhoven MM, van Rosmalen J, et al. Lung function, exercise tolerance, and physical growth of children with congenital lung malformations at 8 years of age. Pediatr Pulmonol 2019; 54:1326.